Antinociceptive potential of methanol leaf extracts of Cissampelos parreira (Linn), Lantana camara (Linn) and Ocimum gratissimum (African basil)
Abstract
The effects of Cissampelos pareira (Linn), Lantana camara (Linn) and Ocimum gratissimum (African basil) leaf extracts on pain have not been biologically determined despite their frequent traditional use in pain management. The present study evaluated the effects of methanol leaf extracts of these three plants on formalin-induced pain in Swiss albino mice. Leaves of C. pareira, L. camara, and O. gratissimum were harvested, cleaned, shade dried, crushed, extracted in absolute methanol and concentrated to dryness. The quantitative phytochemical screening of the three plant extracts was first carried out. Then, the pain assay tests constituted eight groups of five mice each: normal control group, positive control group, negative control group and experimental groups of 50, 100, 150, 200 and 250 mg/kg bw extracts. The animals were administered with various treatments thirty minutes before induction of pain through injection of 0.01 ml of 2.5% formalin solution into the sub-plantar region of the left hind paw. Paracetamol at the dose of 50 mg/kg bw and 5% dimethyl sulfoxide were used as the positive and negative controls respectively. The plant extracts were administered intraperitoneally and orally. Data was analyzed using one-way analysis of variance and unpaired t-test. Phytochemical screening on separate extracts of C. pareira, L. camara, and O. gratissimum revealed fatty acids, phenols, flavonoids and terpenoids. The different dosages of methanol leave extracts of C. pareira, L. camara, and O. gratissimum reduced pain significantly (p˃0.05) in mice. The significant reduction of pain was associated with fatty acids, phenols, flavonoids and terpenoids revealed in the plant extracts.
INTRODUCTION
Pain is a common manifestation of many diseases afflicting millions of people worldwide [1]. It is a phenomenon experienced during disease processes and it runs an acute and chronic course, also referred to as phase one and two or early and late phases, respectively. Acute pain is mild and can last for just a moment, or it might be severe and last for weeks or months. It serves a protective purpose, warning the body of impending danger by activating the “fight or flight” response of the sympathetic nervous system, which often produces signs such as elevated blood pressure, dilated pupils and tenseness of skeletal muscles [2].
Acute pain may also be exhibited through behavioral signs such as crying, moaning, or guarding painful body areas. However, physiologic and behavioral signs are not always evident in acute pain and if present, may last for a short period. With longer exposures to pain, physiologic adaptation may occur, and the body may change its behavior to appear unresponsive because of exhaustion [3].
On the other hand, chronic pain is normally continuous, carries on for at least six months and habitually grows insidiously. It goes on past the course of an acute disease or after tissue healing is over. It has no biological function because it is not a sign of a disease process as it is a disease process itself [4]. The cause of chronic pain is often unknown. Important behavioral and psychological alterations that are seen as a result of chronic pain include depression, sleeping disorders and a tendency to deny pain. The most common types of chronic pain include persistent low back pain, pain associated with cancer, neuralgias, hemiagnosia and phantom limb pain [5].
Conventional approaches used in pain management include acetaminophen (paracetamol) and Non-Steroidal Anti-Inflammatory Drugs (NSAIDs) such as diclofenac and aspirin. Acetaminophen is believed to attenuate pain by inhibiting the activity of the cyclooxygenase enzyme [6]. However, these drugs are accompanied by side effects such as nausea, constipation, skin allergies, liver and renal impairment and cardiovascular disorders [7]. Other alternative methods used for pain relief include plant extracts, decoctions, faith, and acupuncture. The use of botanicals in managing painful conditions has gained first-class awareness, arguably because they are culturally acceptable, give much less or no adverse outcomes, easily accessible and affordable [8].
The use of medicinal plants for thousands of years by indigenous populations has attracted research on their empirical knowledge. Therefore, medicinal plants demand adequate evaluation of their efficacy, safety and mechanism of action [9]. Some herbal extracts that are used as a folklore remedy for pain have been scientifically confirmed. They include Lonchocarpus eriocalyx [10], diphenyl diselenide [11], Clinacanthus nutans [12], and Borreria verticillata [13] among others.
The present study evaluated the antinociceptive effects of methanol extracts of Cissampelos pareira (Linn), Lantana camara (Linn) and Ocimum gratissimum (African basil). Cissampelos pareira is a climber with heart-shaped leathery leaves, 3m tall and has orange fruits. The flowers are small in size with threadlike pedicels. It is common in deciduous bushland and near rock outcrops [14]. Lantana camara is a perennial erect hardy shrub with a powerful odour and grows up to 1 to 3 meters. It occurs in riparian zones and forest edges [15]. On the other hand, O. gratissimum is a local shrub with an erected small plumb and many barnacles, with a height between 1-3 meters high. It is well distributed around villages and along streams [16].
Literature has shown that C. pareira, L. camara and O. gratissimum have traditionally been used in the management of pain [15]. In South America, C. pareira is referred to as “Midwife’s herb since it is used to manage menstrual cramps, uterine hemorrhages after childbirth, eases childbirth and postpartum, because of its intense relaxant effect on smooth muscle. It is prepared as a decoction and administered orally [18; 19]. On the other hand, dried leaves of L. camara are boiled in water and the decoction is used to manage hemoptysis and pulmonary tuberculosis [15]. Finally, leaves of O. gratissimum mixed with hot water have been applied to eyes that are hurting, treat gout and colon pain [20; 17]. The selection of the three plants was based on ethnobotanical information from local herbalists, who acknowledged their use as a folklore remedy for pain among communities living in Embu County, Kenya.
However, the continued use of these plants has not been subjected to scientific evaluation to validate their traditional use as therapeutic agents against pain. Against this background, this study was conceived and designed to determine the antinociceptive potential of the methanol leaf extracts of C. pareira, L. camara and O. gratissimum in mice.
MATERIALS AND METHODS
Plant samples collection and extraction
This study obtained a research permit from the National Commission of Science, Technology and Innovation (NACOSTI), Kenya (Re. No: NACOSTI/P/20/5273). Collection of fresh leaves of C. pareira, L. camara and O. gratissimum was done from their natural habitat in Mbeere North Sub-County, Embu County, Kenya, with the assistance of a local herbalist. The plant samples were dusted, packed in khaki bags and transported to Kenyatta University, Department of Biochemistry, Microbiology and Biotechnology, where the study was undertaken. The botanical identification of the plant samples was performed by an acknowledged taxonomist in the same university Mr. Stephen Mwangi. The respective voucher specimens of the plants were then deposited in the Plant Sciences Departmental herbarium. Specimen voucher numbers were assigned as C. pareira (SNK001), L. camara (SNK002) and O. gratissimum (SNK003), respectively.
Before drying, the plant materials were cleaned using distilled water. Next, they were separately shade-dried at room temperature for three weeks. They were then separately ground into fine powders using an electric mill (Christy and Norris Model 8) and stored at room temperature in well labelled khaki bags awaiting extraction. The collection, processing and extraction of the plant materials were done as per the guidelines formulated by World Health Organization on good herbal processing practices for herbal medicines [21].
Powdered plant samples weighing 800 grams were separately soaked in 1800ml of analytical grade methanol (CAS number 67-56-1; Sigma Aldrich; Germany), agitated and left to stand for 24 hours in a well labelled 2-litre conical flask (Borosil®) and corked. The residue was immersed in methanol again after decantation of the mixture and permitted to stand for another 24 hours at room temperature before decanting again. The obtained mixture was filtered using Whatman No.1 paper [22]. The steps were repeated twice, and the menstruum portions put together. Each of the extracts was then concentrated under reduced pressure and controlled temperature of between 40℃-50℃ using a rotary evaporator (Stuart RE400, Stuart, Germany) [23]. The concentrate was allowed to dry up and thereafter placed in pre-weighed well labelled and sealed specimen bottles. The percentage yields of the plant extracts were determined using the formula described by [24]:
Where, W2 is the weight of the extract and the container, W1 is the weight of the container alone and W0 is the weight of the initial dried sample.
The extracts were then refrigerated at 4℃, awaiting use in bioassay studies and phytochemical analysis.
Liquid chromatography-mass spectrometry (LC-MS) analysis
The quantitative phytochemical screening of the three extracts was done at the International Centre for Insect Physiology and Ecology (ICIPE) laboratories, Kenya. The identification of phytochemicals was performed using Agilent MSD 6120-Triple Quadruple LC-MS with an electrospray source (Palo Alto, CA). The system was controlled using Chemstation software (Hewlett-Parkard). Reversed-phase liquid chromatography was performed on an Agilent Technologies 1200 infinite series, Eclipse plus C18 column, 4.6 x 50 mm, 3.5um (Agilents CA814112) using the following gradient program 0 min, 5% B; 0-5min, 5-50% B; 5-10min, 50-80% B; 10-15min, 80-100% B; 15-25min, 100% B; 25-30min, 5% B; 30-35min, 5% B. The flow rate was constant at 1ml/min; the injection volume was 1.0Ul and data was acquired in a full-scan negative ion mode using a 100 to 1500m/z scan range. The dwell time for each ion was 50ms. The mass spectrometer parameters were as follows: capillary voltage 3.0kV; cone voltage 70V; extract voltage 5V; RF voltage 0.5V; source temperature 110℃; nitrogen gas temperature for desolvation, 380℃; and nitrogen gas flow for desolvation 400L/h. The LC-MS analyzed serial dilutions of Kaempferol standard (1-100 ng/µL) in full scan mode to generate linear calibration curve (peak area verses concentration) with the following equation: [y=6008.9x -5250.3 (R2 = 0.9987)] which served as a basis for external quantification. Most of the compounds in the MS exhibited abundance (M-H) in negative ion mode. The single point external standards analyte response was assumed to be linear over concentration. All the peaks for the analyte were subjected to the same method of quantification. LC-MS in full scan mode generated a linear calibration curve (peak area verse concentration) with the following equation: [y=6008.9x-5250.3 (R2 = 0.9987)] which served as a basis for external quantification. A mass of 1mg/mL of each sample was reconstituted in LC-MS methanol from Tedia. Inc USA and 20µl injected into as aforementioned. Pentacyclic triterpenoids present in the extracts were identified using METLIN metabolite data base and literature precedent and quantified using griseofulvin internal standard.
Gas chromatography-mass spectrometry (GC-MS) analysis
One milligram of the dried crude methanol leaf extracts of C. pareira, L. camara and O. gratissimum were dissolved in 1mL dichloromethane (sigma Aldrich GC-grade). The samples were vortexed for 30 seconds and sonicated in an ultra-bath for 15 minutes before centrifugation at 14,000 rpm for 5 minutes. The supernatants were passed through anhydrous Na2SO4 to remove moisture. The resultant stock solutions (1mg/mL) were used to prepare experimental samples whose final concentrations were 100ng/μL. Each sample was prepared in triplicate.
Each sample was analyzed using GC-MS (7890/5975 Agilent Technologies, Inc., Beijing, China), consisting of a Gas Chromatography interfaced to a Mass Spectrometer instrument. The GC-MS was equipped with an HP-5MS (5% phenyl methyl siloxane) low bleed capillary column of 30m length, 0.25mm diameter and 0.25μm film thickness. The GC-MS used a detection with ionization energy of 70Ev. The carrier gas used was helium (99.99%) at a constant flow rate of 1.25ml/min in split mode. The injector and mass transfer line temperature were set at 250℃ and 200℃, respectively, and an injection volume of 1μl was employed. The oven temperature was programmed from 35°C for 5 minutes, with an increase of 10°C/min to 280°C for 10.5 minutes, then 50°C/min to 285°C for 29.9 minutes with a run time of 70 minutes. The mass spectrometry operating parameters were as follows: ionization energy, 70eV; ion source temperature, 230°C; solvent cut time, 3.3 min; relative detector gain mode; scan speed, 1666μ/sec; scan range of 40-550m/z and the interface temperature of 250°C. Identification was based on the molecular structure, molecular mass and calculated fragments. Interpretation on mass spectrum GC-MS was conducted using the database of National Institute of Standard and Technology (NIST) database, which has more than 62,000 patterns. The name, molecular weight and structure of the components of the test materials were ascertained. Library-MS searches using NIST Mass spectral library NIST 05, Chemecol.l, NIST 11 and Adams 2.l databases. NIST mass spectral search program Version 2.0 was used for characterization purposes in the GC-MS data system. The area was used for quantification based on the amount of internal standard added.
Experimental animals
The present study used 240 Swiss albino mice of either sex, aged between 6-8 weeks and weighing 20-25 grams. The mice were obtained from Kenya Medical Research Institute (KEMRI) Nairobi, Kenya, upon ethical approval by the Egerton University Research Ethics Committee, Kenya approval number EUREC/APP/101/2020. The mice were housed in the animal breeding facility in the Department of Biochemistry, Microbiology and Biotechnology at Kenyatta University. The animals were kept in standard cages throughout the study, maintained under room temperature and humidity (55±5%) with 12 hours’ daylight for seven days to acclimatize with the laboratory conditions [25].
The mice were fed on standard rodent pellet diet and were allowed access to water ad libitum [26]. Before conducting the bioassays, the mice fasted for 12 hours. Bio-screening was conducted according to the internationally accepted procedures and ethical guidelines for evaluating efficacy and safety of herbal medicines in animal models [27; 28].
Experimental design and bioassay
This study adopted a completely randomized controlled study design, from which an experimental design was formulated. First acute oral toxicity assay was carried out according to OECD protocol [29]. The acute toxicities of C. pareira, L. camara and O. gratissimum used doses of 1000 and 2000mg/kg bw. After administration of the extract, the animals were under close observation continuously for 1 hour and intermittently for 4 hours and thereafter once every 24 hours for the 14 days. During this period, observations such as mortality, neurological behavior, reduced locomotion and dullness were noted.
Five dose levels were selected for antinociceptive assays (50, 100, 150, 200 and 250mg/kg bw) of the studied plant extracts based on a prior pilot study. Thereafter, Swiss albino mice were randomly divided into eight groups of five mice each (n=5). Group I (Normal control) mice were intraperitoneally administered with 5%DMSO solution only. Group II (Negative control) mice were intraperitoneally administered with 5% DMSO solution 30 minutes prior to pain induction and no treatment was administered. Group III (Positive control) mice were intraperitoneally administered with reference drug (Paracetamol) at a dose of 50mg/kg bw 30 minutes prior to pain induction. Groups IV, V, VI, VII and VIII mice were intraperitoneally treated with extract dose levels of 50, 100, 150, 200 and 250mg/kg bw respectively, 30 minutes before induction of pain. The same experimental setup was done with orally administered treatments.
Antinociceptive activity assay
The antinociceptive effects of the three methanol extracts were determined using formalin-induced paw licking model in mice as described by [30]. Half an hour after administering the reference drug (Paracetamol) and methanol extracts, the pain was initiated by administering 0.05ml of 2.5% formalin into the sub-plantar region of the left hind paw and the pain behavior observed and recorded. In addition, the time in seconds that the mouse spent lifting, licking or biting the injected paw was counted using a digital stopwatch (3D Max 2011 Model) and recorded as the measure of pain [31]. In all groups, the mice were individually placed in a transparent plexiglass observation chamber with mirrors placed on two sides of the chamber to observe the nociceptive behavior from all angles. All injections were done using 30-gauge needles.
The nociceptive behavior was monitored and recorded according to the response pattern described by [31]. Two distinct periods of nociceptive behavior were identified and scored separately as early phase and late phase. The early phase was recorded 1-5 minutes after formalin injection. The late phase was recorded 15-30 minutes after formalin injection. The fifth and the fifteenth minute duration was the remission period with minimal nociceptive behavior [32]. The percentage paw licking inhibition was determined using the formula described by [33].
Where, N- The negative control group value for each phase, T-The treated group value for each phase
Statistical analysis
Quantitative experimental data on paw licking, biting, or lifting time obtained from all the animals in various treatment groups were recorded and tabulated in a Microsoft Excel spreadsheet and transferred to a Minitab spreadsheet. The data were subjected to descriptive statistics and presented as Mean ± Standard Error of Mean (SEM) and then checked for normality using the Kolomogorov-Smirnov test. Analysis was done using One-Way Analysis of Variance (ANOVA) followed by Tukey’s post hoc for pairwise separation and comparison of means. In addition, unpaired Student t-test was used to compare the antinociceptive activities of orally and intraperitoneally administered treatments. Analysis of the data was done using Minitab® Version 17 software. Statistical significance was set at 95% confidence interval. Results were presented in tables and figures.
RESULTS
Percentage yield of the plant extracts
The final yields of L camara and C. pareira were blue-black pastes while that of O. gratissimum was a brown, black solid. The percentage yield from C. pareira, L. camara and O. gratissimum leaf extracts were 21, 24 and 23%, respectively.
Quantitative phytochemical analysis
The LC-MS analysis of the leaf extracts of C. pareira, L. camara and O. gratissimum revealed the presence of phytoconstituents such as caffeic acid, cissamine, quercetin, luteolin, apigenin, among others (Table 1). On the other hand, the GC-MC screening of the three plant extracts demonstrated the presence of α-pinene, cymene, limonene, oleic acid, among others (Table 1).
Table 1. Phytochemicals revealed in the methanol leaf extracts through LC-MS and GC-MS analysis.
Acute toxicity
No deaths were reported following oral administration of the studied plant extracts at the dose of 1000 and 2000mg/kg bw. In addition, no clinically abnormal signs were observed.
In vivo antinociceptive activities of oral and intraperitoneal administration of methanol leaf extract of Cissampelos pareira
In general, both orally and intraperitoneally administered methanol leaf extract of C. pareira significantly reduced paw licking time in a dose-independent fashion in the early and late phases following formalin-induced nociception in mice. In addition, the antinociceptive activities exhibited a decrease in paw licking time or raised percent paw licking inhibition following formalin-induced pain in mice (Table 2 and 3). It was noted that the highest analgesic activity in both phases was at the extract dose level of 150mg/kg bw (Table 2). At this dose, the antinociceptive effect was statistically similar to that of the reference drug (paracetamol) (p>0.05; Table 2). The extract exhibited the least antinociceptive effect at the dose of 250mg/kg bw in both phases (Table 2).
In the early phase, the range of pain inhibition of the orally administered methanol leaf extract of C. pareira at the five dose levels was between 31.75 and 61.02%. It was noted that the analgesic effect of the extract dose levels of 50, 100, 150, 200 and 250mg/kg bw was statistically similar to that of the standard drug (p>0.05; Table 2). Further, it was observed that there was no significance difference in the antinociceptive activity of the extract dosages of 100, 150 and 200mg/kg bw (p>0.05; Table 2). In addition, the effect of the extract at the doses of 50, 100, and 200mg/kg bw was statistically similar (p>0.05; Table 2).
In the late phase, the five extract dose levels of 50, 100, 150, 200 and 250mg/kg bw reduced the formalin-induced pain in mice by 27.51, 37.21, 40.92, 26.63 and 10.68% respectively (Table 2). The percent paw licking inhibition of the extract at dose levels of 50, 100, 150 and 200mg/kg bw were not significantly different and comparable to that of the standard drug (p>0.05; Table 2). In addition, the efficacy of the extract at the doses of 50, 200 and 250mg/kg bw was statistically similar to each other (p>0.05; Table 2).
In the early phase, the intraperitoneally administered methanol leaf extract of C. pareira inhibited pain by 59.73, 70.90, 64.73, 40.21 and 35.98% at the extract dose levels of 50, 100, 150, 200 and 250mg/kg bw respectively (Table 3). Furthermore, it was observed that the highest analgesic effect was observed at the extract dosage of 100mg/kg bw while the least effect was at the extract dose of 250mg/kg bw in both phases (Table 3). Notably, the extract dose levels of 50, 100 and 150mg/kg bw as well as the reference drug evoked nociceptive inhibitions that were comparable to each other (p>0.05; Table 3). On the other hand, the efficacy exhibited by the extract dosages of 200 and 250mg/kg bw was statistically similar to each other (p>0.05; Table 3).
In the late phase, the range of antinociceptive action of the C. pareira extract at the five dose levels ranged between 16.23 and 64.90% (Table 3). It was noted that the extract doses of 50, 200 and 250mg/kg bw caused statistically similar analgesic effect (p>0.05; Table 3), but the effect was significantly lower than that of the standard drug (p<0.05; Table 3). Further, the percent inhibitions of nociception of the extract dose levels of 100 and 150 mg/kg bw were comparable to the effects caused by the reference drug (p>0.05; Table 3).
Table 2. Antinociceptive effect of orally administered methanol leaf extract of C. pareira on formalin-induced pain in mice.
Table 3. Antinociceptive effect of intraperitoneally administered the leaf extract of C. pareira on formalin-induced pain in mice.
Comparison between antinociceptive effects of orally and intraperitoneally administered C. pareira extract.
Upon pairwise comparison of the orally and intraperitoneally administration of methanol leaf extract of C. pareira, it was observed that the potency of the intraperitoneally administered extract was significantly higher than that of orally administered extract in the early phase at the extract dose of 50 and 100mg/kg bw (p<0.05; Figure 1).
On the other hand, the effects of the orally and intraperitoneally administered extract in the late phase were statistically similar (p>0.05) except at the doses of 100 and 250mg/kg bw (p<0.05; Figure 2).
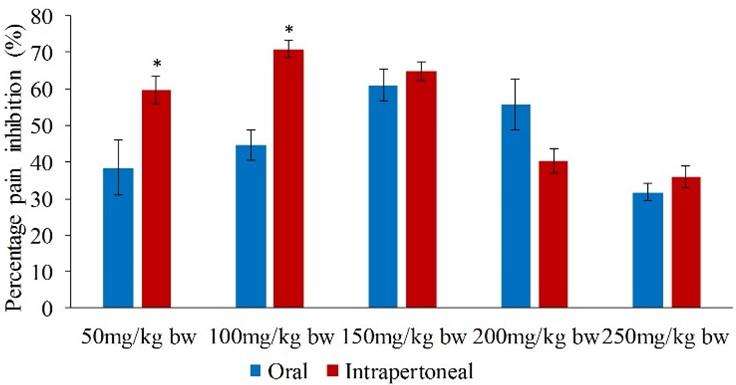
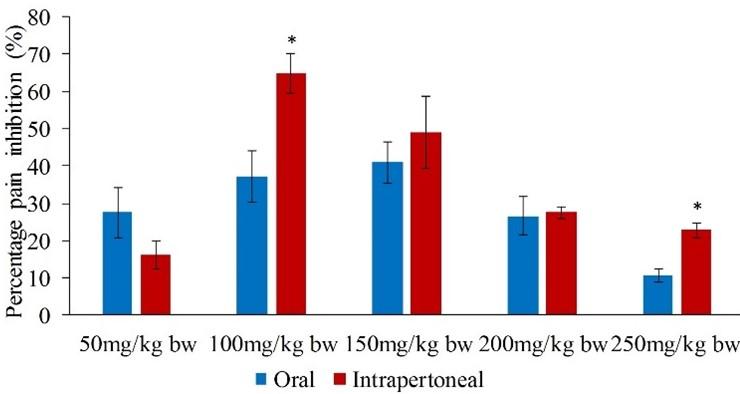
In vivo antinociceptive activities of oral and intraperitoneal administration of methanol leaf extract of L. camara
Overall, the orally and intraperitoneally administered methanol leaf extract of L. camara inhibited nociception in a dose-independent manner in both the early and late phases following formalin-induced nociception in mice (Table 4 and 5). In addition, the analgesic effects were demonstrated by reduced paw licking time or increased percent paw licking inhibitions on formalin-induced pain in mice.
In both phases, the orally administered extract revealed the highest pain inhibition at the extract dose of 100mg/kg bw, while the lowest pain inhibition was at the extract dose of 250mg/kg bw (Table 4). In the early phase, the oral administration of the extract reduced pain by between 39.86 and 67.02% (Table 4). The potency of the extract doses of 50, 150, 200 and 250 was statistically similar to that of the reference drug (paracetamol) (p>0.05; Table 4).
In the late phase, the extract dose levels of 50, 100, 150, 200 and 250mg/kg bw inhibited formalin-induced pain in mice by 34.22, 41.27, 36.51, 27.16 and 22.05% respectively (Table 4). Remarkably, the five extract dose levels and the standard drug evoked efficacies that were statistically similar to each other (p>0.05; Table 4).
In the early phase, the five doses (50, 100, 150, 200 and 250mg/kg bw) of intraperitoneally administered extract of L. camara reduced the formalin-induced pain by 62.12, 73.72, 79.89, 71.43 and 64.73% respectively (Table 5). The highest percentage inhibition of pain was observed at 150mg/kg bw, while the dose of 50mg/kg bw revealed the least inhibition (Table 5). It was further noted that the effect of the five extract doses was statistically similar compared to that of paracetamol (reference drug) (p>0.05; Table 5).
In phase two of the test period, the inhibitions of nociception by the five intraperitoneally administered extract doses of L. camara ranged between 21.69 and 62.05% (Table 5). In this phase, the lowest analgesic activity was observed at the extract dose level of 250mg/kg bw. At the same time, the extract dosage of 100mg/kg bw generated the greatest potency, which was comparable to the effect of the standard drug (p>0.05; Table 5). Notably, the effect of the extract at the dose levels of 150, 200 and 250mg/kg bw showed no significant difference (p>0.05; Table 5). Furthermore, the extract’s efficacy at the dose levels of 50, 150, 200 and 250mg/kg bw was significantly lower than that of the standard drug (p<0.05; Table 5).
Table 4. Antinociceptive effect of orally administered methanol leaf extract of L. camara on formalin-induced pain in mice.
Table 5. Antinociceptive effect of intraperitoneally administered leaf extract of L. camara on formalin-induced pain in mice.
Comparison between antinociceptive effects of orally and intraperitoneally administered L. camara extract.
In phase one, it was observed that the potency of the intraperitoneally administered extract was significantly higher compared to that of orally administered extract at the doses of 150 and 250mg/kg bw (p<0.05; Figure 3).
Nevertheless, in the second phase, the effects of the intraperitoneally and orally administered extracts were comparable to each other (p>0.05; Figure 4) except at the extract dose of 150mg/kg bw (p<0.05; Figure 4).
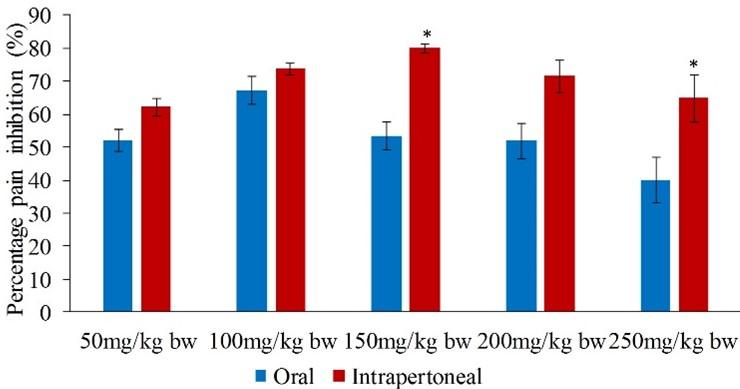
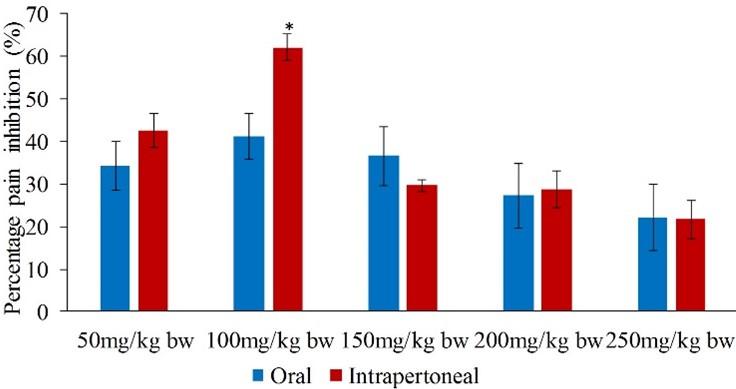
In vivo antinociceptive activities of oral and intraperitoneal administration of methanol leaf extracts of O. gratissimum
Generally, the potency of the orally and intraperitoneally administered methanol leaf extracts of O. gratissimum was dose-independent in the first and second phases following formalin-induced nociception in mice (Table 6 and 7).
The extract inhibited pain in the early phase between 35.27 and 51.50% (Table 6). It was noted that the least percentage inhibition of pain was at the dose of 250mg/kg bw while the highest percentage inhibition of pain was attained at the dose of 150mg/kg bw. It was further observed that the effects of all the extract dose levels, as well as the reference drug, were comparable to each other (p>0.05; Table 6).
In the second phase, the extract dose levels of 50, 100, 150, 200 and 250mg/kg bw decreased formalin-induced pain in mice by 11.89, 22.22, 28.22, 20.11 and 14.29% respectively (Table 6). The highest analgesic effect was seen at the extract dosage of 150mg/kg bw, while the extract dose of 50mg/kg bw had the lowest antinociceptive activities (Table 6). Furthermore, the effect of the extract doses of 50, 100, 200 and 250mg/kg bw was not significantly different (p>0.05; Table 6). However, the effect of the standard drug was significantly higher than that of the extract at the five dose levels tested (p<0.05; Table 6).
The intraperitoneally administered O. gratissimum extract at the doses of 50, 100, 150, 200 and 250mg/kg bw significantly reduced formalin-induced nociception by 37.21, 58.20, 53.26, 49.21 and 40.21% respectively, in the first phase of the test period (Table 7). The least efficacy was observed at the extract dose of 50mg/kg bw in both early and late phases. It was noted that the peak of analgesic effect was observed at the extract dose of 100mg/kg bw (Table 7). Additionally, it was observed that the efficacy of the extract dose levels of 50, 200 and 250mg/kg bw was not statistically significant (p>0.05; Table 7). The effect of the extract at the five dose levels was significantly lower than that of the reference drug (p<0.05; Table 7).
In the second phase, the range of pain inhibition caused by the extracts of O. gratissimum was between 16.26 and 38.25% (Table 7). The extract dose of 150mg/kg bw attained the highest analgesic effect (Table 7). It was observed that the potency of the extract at the dose levels of 150, 200 and 250mg/kg bw was not statistically significant (p>0.05; Table 7). However, the effect of the extract at the doses of 50, 100 and 150 was significantly different (p<0.05; Table 7). Interestingly, all the extract dosages demonstrated antinociceptive effects that were significantly lower than the effects caused by the standard drug (p<0.05; Table 7).
Table 6. Antinociceptive effect of orally administered leaf extract of O. gratissimum on formalin-induced pain in mice.
Table 7. Antinociceptive effect of intraperitoneally injected leaf extract of O. gratissimum on formalin-induced pain in mice.
Comparison between antinociceptive effects of orally and intraperitoneally administered O. gratissimum extract.
It was observed that the efficacies of the orally and intraperitoneally administered extract were statistically similar (p>0.05) in the first phase of the experimental period at all the tested dose levels except at the dose of 100mg/kg bw (p<0.05; Figure 5).
Likewise, it was noted that in the late phase, the effects of the orally and intraperitoneally administered extract were comparable to each other at the extract doses of 50, 100, 150 and 200mg/kg bw (p>0.05; Figure 6).
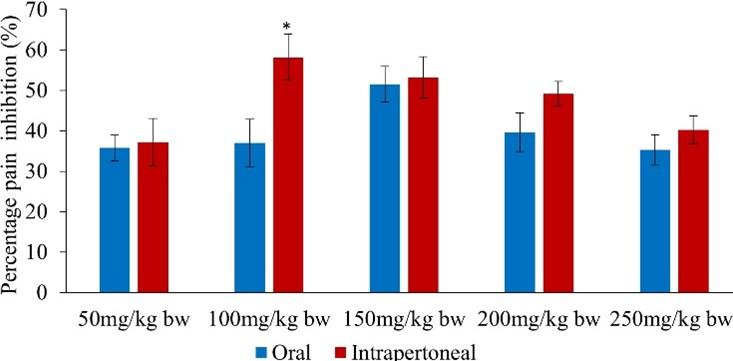
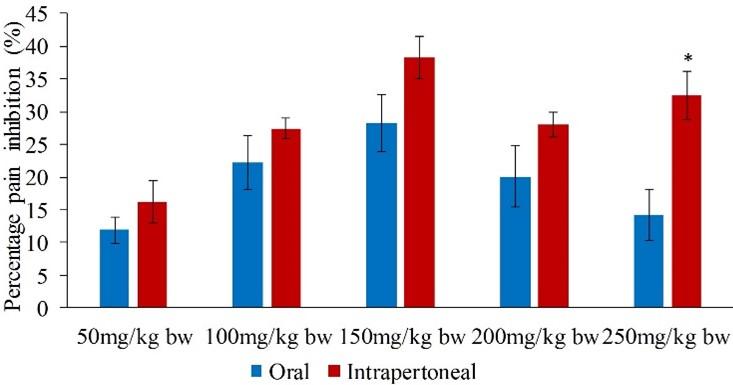
Comparison of antinociceptive effects of methanol leaf extracts of C. pareira, L. camara and O. gratissimum
It was observed that the effects of the three orally administered methanol extracts of C. pareira, L. camara and O. gratissimum were comparable to each other at the dose levels of 150, 200 and 250mg/kg bw in the early phase (p>0.05; Figure 7). Notably, in the same phase, the effect of oral administration of L. camara extract at the doses of 50 and 100mg/kg bw the L. camara extract was significantly higher compared to those of C. pareira and O. gratissimum (p<0.05; Figure 7).
In the second phase, the analgesic effects of the orally administered methanol extracts were statistically similar at the dose levels of 100, 150, 200 and 250mg/kg bw (p>0.05; Figure 8). Nevertheless, the effect of L. camara extract at the dose level of 50mg/kg bw was significantly higher than that of O. gratissimum extract (p<0.05; Figure 8).
On the other hand, intraperitoneally administered extract of O. gratissimum at the dose of 50mg/kg bw, caused analgesic effects that were significantly lower than those of L. camara and C. pareira in the early phase (p<0.05; Figure 9). It was also noted that antinociceptive effect of L. camara extracts at the dose of 100mg/kg bw was significantly higher than that of O. gratissimum (p<0.05; Figure 9). Besides, the O. gratissimum extract at the dose of 200mg/kg bw exhibited higher antinociceptive effect than that of C. pareira extract (p<0.05; Figure 9). Further, effect of L. camara extract at the doses of 150 and 250mg/kg bw was significantly higher than those of C. pareira and O. gratissimum extracts (p<0.05; Figure 9).
In the late phase, the antinociceptivevity of L. camara extract at the dose level of 50mg/kg bw was significantly higher than those of C. pareira and O. gratissimum extracts (p<0.05; Figure 10). It was also observed that at the extract dose of 100mg/kg bw the potency of O. gratissimum extracts was significantly lower than those of L. camara and C. pareira extracts (p<0.05; Figure 10). However, at the extract dose of 250mg/kg bw, the effect of O. gratissimum extract was significantly higher than those of L. camara and C. pareire extracts (p<0.05; Figure 10). In addition, it was observed that at the extract dose level of 150mg/kg bw, C. pareira extracts exhibited a higher antinociceptive effects than L. camara extract (p<0.05; Figure 5c). Further, at the dose level of 200mg/kg the effects of the three studied extracts bw were statistically similar (p>0.05; Figure 10).
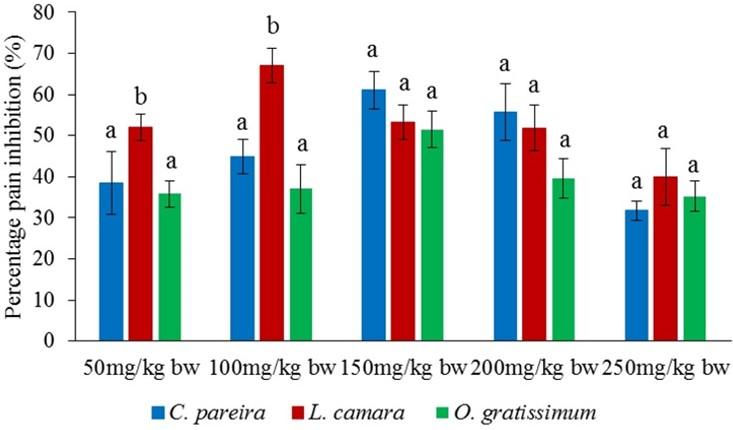
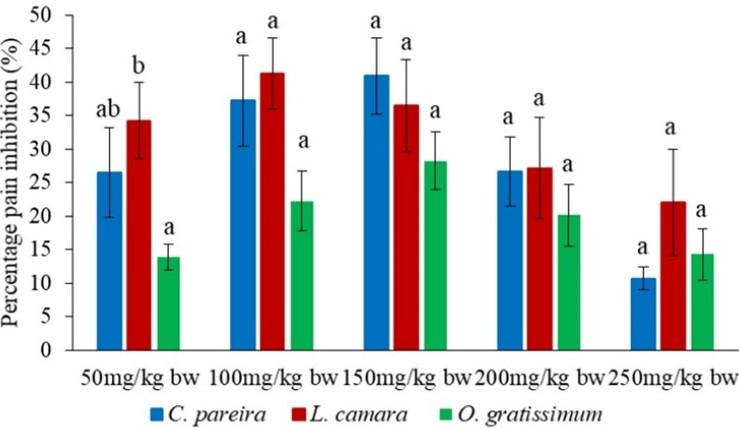
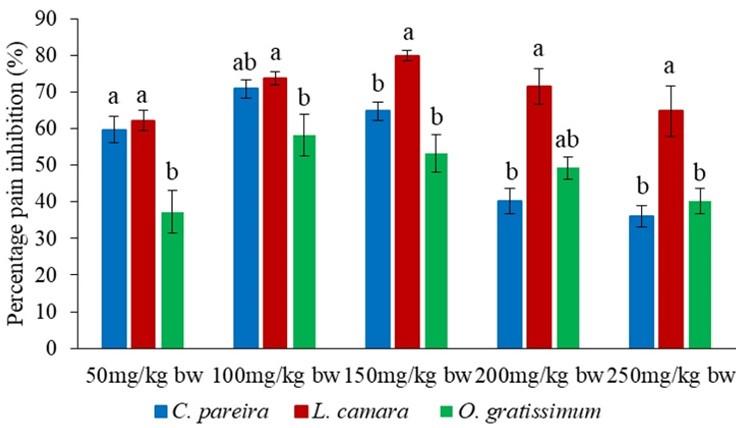
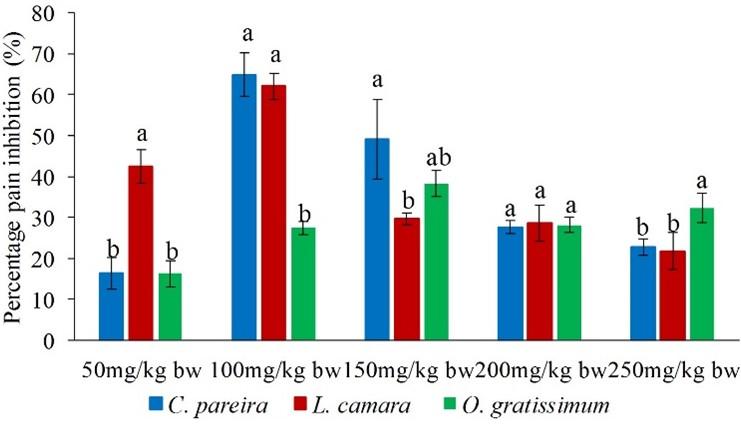
DISCUSSION
The current study was designed to evaluate the analgesic potential of methanol leaf extracts of C. pareira, L. camara and O. gratissimum in mice. Based on the acute toxicity results the studied plant extracts were found to be safe at the tested doses. The oral and intraperitoneal administration of the three methanol leaf extracts exhibited potent analgesic effects in vivo. The antinociceptive activities of the extracts was manifested by reduction in paw licking time, which was expressed as percent paw licking inhibition. These findings correlated with earlier studies undertaken on medicinal plants using animal models.
A study by [34] on dichloromethane root bark extract of Carissa edulis reported antinociceptive activity in early and late phases of formalin-induced pain in mice model. In yet another study, [35] demonstrated analgesic effect of Caesalpinia volkensii (Harms) in the early and late phases of formalin-induced nociception in mice models.
In the current study, methanol extracts of C. pareira, L. camara and O. gratissimum caused antinociceptive effects of between 31.75 and 79.89% in the early phase, and between 10.68 and 64.90% in the late phase. These findings are consistent with those reported on Harrisonia abyssinica, extract which exhibited analgesic activity of between 39.73 and 81.13% in the early phase, and between 15.92 and 69.84% in the late phase in mice [36].
The extract dose ranges used in the current study were within the scale used by previous studies on analgesic effects of medicinal plants in animal models. For example, a study by [37] on analgesic activity of dichloromethane root extract of Clutia abyssinica in Swiss albino mice, used doses of 50, 100 and 150mg/kg bw. Similarly, a study by [38] on the analgesic potential of dichloromethane leaf extracts of Eucalyptus globulus and Senna didymobotrya in mice used doses of 25, 50, 100, 150, 200 and 250mg/kg bw.
In the present study, GC-MS and LC-MS analysis were performed. This was informed by the fact that, GC-MS is useful for monitoring highly hydrophobic and volatile compounds. On the other hand, LC-MS is suitable for the analysis of large, ionic, thermally unstable and non-volatile compounds. It also allows the analysis of polar compounds in water samples [39].
It was noted that the intraperitoneally administered extracts were significantly more effective than the orally administered extracts. The higher analgesic activities caused by the intraperitoneally administered extracts may have been due to high concentrations of bioactive compounds in the peritoneal cavity which bypassed the fast pass metabolic effect in the liver or gut. According to [40], when a drug is orally administered, it passes through the fast pass effect in the liver or gut, where it is absorbed, metabolized and distributed before it reaches the systemic circulation. A previous study performed on Rheedia longifolia LAE reported that it was effective when administered orally and intraperitoneally, though the intraperitoneally administered extract had the highest analgesic effect [41].
The three methanol leaf extracts caused a non-dose-dependent antinociceptive effect. Both phases of formalin-induced pain imply that the highest analgesic effects were observed at lower doses, after which the activities decreased steadily at higher dose levels. This scenario could be ascribed to the saturation of bioactive compounds at the binding sites of the receptors involved in antinociceptive mechanisms at lower extract doses. The phenomenon may also be linked to antagonistic action of the antinociceptive phytocompounds with other phytoconstituents present in the methanol leaf extracts. This possibly suggests that at higher doses, the activity of phytocompounds not associated with pain may have been antagonistic to those with antinociceptive properties.
These results correlated with previous findings, which reported non-dose-dependent antinociceptive effects. For example, a study by [42] while evaluating antinociceptive effects of Acacia mellifera extracts showed non-dose-dependent response. Similarly, a study by [43] on antinociceptive effects of Maytenus obscura demonstrated a non-dose-dependent response.
In addition, the least antinociceptive activity of O. gratissimum extract was recorded at the dose level of 50 mg/kg bw. Therefore, it was possible that, at lower concentrations the bio-active principles in the extracts may have been metabolized, cleared or inactivated at a faster rate. Furthermore, the strength of the bioactive molecules, at lower dose levels, may have had inadequate pharmacological action [44].
There was pain inhibition during the early phase as well as the late phase of formalin-induced pain. However, the pain inhibition in both phases was not equal; the three methanol extracts had higher analgesic effects in the early phase than in the late phase. This suggests that the plant extracts contain centrally and/or peripherally acting analgesic bioactive compounds. The activities in the early phase may be attributed to fast diffusion of the active principles across the cell membranes into the peritoneal cavity, thereby causing the faster onset of analgesic effects. Besides, the phytocompounds contained in the methanol extracts of C. pareira, L. camara and O. gratissimum, might have applied antinociceptive action first-hand in the absence of biotransformation. Additionally, the methanol plant extracts used in this study may have had high concentrations of opioid-like analgesic phytoconstituents that exerted a central analgesic effect that was observed in the early phase.
The peripheral analgesic effects (inflammatory pain) may compare to NSAIDs, which produce their effect by blocking the synthesis of prostaglandins [45]. On the other hand, the knowledge of the mechanism of action of paracetamol is scarce. Still, it is believed that it acts by blocking prostaglandins’ production, hence exerting consequent effects [46]. Alternative mechanisms of action of paracetamol include inhibition of L-arginine-nitric oxide (NO) pathway mediated through substance P or N-methyl-D-aspartate (NMDA), reinforcement of descending inhibitory serotonergic pain pathways and active paracetamol metabolites that have effects on cannabinoid receptors [46].
Studies have shown that various medicinal plant species exert anti-nociceptive effects through the phytochemicals they possess such as alkaloids, flavonoids, terpenoids and saponins [47]. In this study, the LC-MS and GC-MS analysis revealed different categories of phytochemicals such as phenylpropanoid compounds, flavonoids, terpenoids, fatty acids and alkaloids. These phytocompounds included luteolin, caffeic acid, quercetin, apigenin, salvigenin, vitexin, eugenol, limonene and oleanolic acid. These phytocompounds, present in the studied plant extracts, could have mimicked the mode of action of paracetamol as well as NSAIDs to confer their analgesic potential.
Earlier research has attributed antinociceptive properties of the extracts to the presence of phytocompounds. A study conducted on extracts and fractions of Astragalus hamosus revealed flavonoids, saponins, terpenes, alkaloids, tannins and phenols. The analgesic activity of the extract was attributed to these phytocompounds [48]. The terpenoid α-pinene was revealed through the GC-MS analysis of the plant extracts used in this study. A related study on evaluating the antinociceptive potential of α-pinene in rats and mice showed that its effects are equivalent to those of naloxone [49].
Phytochemical screening of the plant extracts in this study showed the presence of limonene. The terpenoid limonene has been reported to have a significant antinociceptive effect in different models of nociception without opioid receptor stimulation [49]. However, the antinociceptive effect of limonene may be related to this compound’s appreciable anti-inflammatory activity, which can decrease cell migration and cytokine release, besides possessing a potent antioxidant effect [50].
Eucalyptol (1,8-cineol) was another compound present in L. camara extracts. Studies have shown that low doses of 1,8-cineol were almost equivalent to morphine in rats, displaying antinociceptive action at spinal and supraspinal levels, whereas in mice a poor supraspinal effect was exhibited [49]. It has been reported that the potent anti-inflammatory action of eucalyptol can be associated with the excellent peripheral analgesic effect. Several studies have shown that eucalyptol confers its analgesic effect through the inhibition of the cyclooxygenase (COX) and suppression of arachidonic acid metabolism and cytokine production such as tumor necrosis factor-α and interleukin-1β (TNF-α and IL-1β) [49].
The analgesic activities of the three studied extracts could have been due to the mechanism of action of cymene. A previous study evaluating antinociceptive effects of cymene, reported that it had both peripheral and central antinociceptive [51]. Research has further shown that cymene lowers TNF-α; this requires the involvement of the descending pain-inhibitory mechanism through the opioid system activation [51]. Furthermore, it has been found that cymene is metabolized to carvacrol and hydroxy-carvacrol in some rodent species [52]. Carvacrol possesses antinociceptive activity associated with the inhibition of prostaglandin synthesis [53]. Thus, cymene could act as a pro-drug that, when bio-transformed into carvacrol, may have its antinociceptive effects enhanced.
The phytochemical screening of methanol extracts of L. camara and O. gratissimum revealed camphor as one of the phytocompounds. A previous study aimed at exploring analgesic effects of camphor, found that it significantly decreased the pain threshold of rats and reduced macrophage and lymphocyte infiltration. In addition, camphor was reported to inhibit interleukin -6 (IL-6), interleukin-8 (IL-8), TNF-α, and IL-1β expression by repressing nuclear factor kabba beta cells (NFκβ) stimulation [54].
The GC-MS analysis of C. pareira and O. gratissimum extracts in the current study also revealed essential fatty acids such as oleic, palmitic and linoleic acids. Previous studies conducted on fatty acids have revealed that their antinociceptive mechanism occurs through inhibition of both first and second phases of pain equally as well as inhibition of prostaglandin synthesis [55]. It has also been reported that essential fatty acids are associated with changes in nitric oxide (NO) production, neutrophil influx and expression of pro-inflammatory proteins such as the inducible nitric oxide synthase (iNOS) and cyclooxygenase-2 (COX 2) [55].
Linoleic acid has been shown to inhibit the formation of prostaglandins and leukotrienes from arachidonic acid, while palmitic acid and derivatives decrease thermal nociception in animal models [56]. Further, it has been shown that palmitic acid, through granulation in mast cells, reduces the release of histamine from the mast and reduced release of nitric oxide and inhibits the expression of the cyclooxygenase-2 enzyme, thereby resulting in analgesic effects [57]. Studies have also shown that oleic acid inhibits the release of calcium from intracellular resources and protects the brain against inflammatory cytokine injuries (TNF-α, IL-6, IL-1β) as well as inflammatory eicosanoids (PGE2) [58].
The LC-MS analysis of L. camara extract revealed the presence of caffeic acid and martynoside. These phenylethanoid and phenylpropanoid compounds have been shown to influence on the metabolism of arachidonic acid and histamine release [59]. Such metabolites can inhibit lysosomal enzyme secretion and arachidonic acid release from membranes by inhibiting lipoxygenase, cyclooxygenase and phospholipase A2. Further, arachidonic acid suppression by inflamed cells could reduce endoperoxides, prostaglandins, prostacyclin, and thromboxanes from the lipoxygenase pathway as well as leukotrienes from the cyclooxygenase pathway [60].
In the present study, the three methanol leaf extracts were shown to contain apigenin and luteolin, while quercetin was shown in O. gratissimum extract. A previous study aimed at exploring antinociceptive activities of Salvia officinalis revealed apigenin, luteolin and quercetin as some of its major constituents. The results showed that the extract significantly decreased the pain threshold in animal models. Furthermore, apigenin and luteolin were reported to reduce inflammatory pain receptors by inhibiting the accumulation of receptors and signaling cascades [61]. On the other hand, quercetin was reported to inhibit lipoxygenase and cyclooxygenase activities [62]. The LC-MS analysis of the methanol leaf extract of C. pareira revealed the presence of cissamine. This alkaloid has been reported to possess anti-nociceptive properties through inhibition of prostaglandin-like mediators. In addition, the analgesic activities of alkaloids have been attributed to the reduction in membrane phospholipase activity and synthesis of pain and inflammation mediators such as cytokines, eicosanoids, leukotrienes and prostaglandins. Further, antinociceptive effects of alkaloids cause inhibition of both the spinal reflex and supraspinal centers [63].
It was apparent that the methanol leaf extract of L. camara showed the most effective analgesic effects compared to C. pareira and L. camara extracts. Notably, the methanol leaf extract of L. camara was found to be endowed with eucalyptol and cymene, which have been reported to possess immense analgesic properties as earlier aforementioned.
The present study revealed that the three methanol leaf extracts contained flavonoids, phenolic acids, terpenoids, fatty acids and alkaloids that have been shown to possess analgesic activity. This observation suggests that the analgesic activity of the extract could have been as a result of the action of one of the bioactive compounds present in the extract or the interaction of the different compounds, which have been shown to have different mechanisms of action [34].
CONCLUSION
The studied plant extracts of C. pareira, L. camara and O. gratissimum exerted substantial analgesic effects in mice. The analgesic effects of the three extracts were attributed to the phytochemical present. Therefore, this study recommends that the methanol extracts of the three plants be used as potential alternative antinociceptive agents. In addition, C. pareira, L. camara and O. gratissimum plants have shown prospects for future drug development studies.
ACKNOWLEDGMENT
Authors wish to thank Kenyatta University for permitting the use of their laboratories for experimentation as well as herbarium facilities. The authors also appreciate Enock Wambugu’s technical support during experimentation at the Biochemistry, microbiology and biotechnology Departmental laboratories, Kenyatta University. In addition, the authors are grateful to Kenya Medical Research Institute (KEMRI) for availing experimental animals, Kenyan Government Chemist and the International Centre of Insect Physiology and Ecology (ICIPE) for allowing us to use their laboratories to carry out GC-MS and LC-MS analysis of the plant extracts.
AUTHOR CONTRIBUTIONS
Kimuni Susan Nyawira, Gitahi Stephen Maina, Njagi Eliud Mwaniki and Ngugi Mathew Piero were involved in the conception and design of the experiments. Kimuni Susan Nyawira and Gitahi Stephen Maina collected the samples. Kimuni Susan Nyawira, Gitahi Stephen Maina, Njagi Eliud Mwaniki and Ngugi Mathew Piero performed experiments and analyzed data. Kimuni Susan Nyawira contributed to drafting the article. Gitahi Stephen Maina, Njagi Eliud Mwaniki and Ngugi Mathew Piero contributed to revising it critically for important intellectual content. Ngugi Mathew Piero made the final approval of the version to be published.
CONFLICTS OF INTEREST
There is no conflict of interest among the authors.
References
- [1]Rang H, Dale M, Ritter J, Flower R, and Henderson G. Pharmacology, 7th edition, Elsevier Churchill Livingstone, Edinburgh, UK; 2011.
- [2]Caspersen C, Powell K, and Christenson G. Physical activity, exercise, and physical fitness: definitions and distinctions for health-related research. Public health rep 1985; 100 (2), 126-131.
- [3]Polomano, R., Fillman, M., Giordano, N., Vallerand, A., Nicely, K., and Jungquist, C. Multimodal analgesia for acute postoperative and trauma-related pain. AJN The American Journal of Nursing, 2017; 117(3), S12-S26.
- [4]Phillips, K., and Clauw, D. Central pain mechanisms in chronic pain states–maybe it is all in their head. Best practice and research Clinical rheumatology, 2011; 25(2), pp.141-154.
- [5]Woessner, J. (2006). Overview of pain: Classification and concepts. Weiner’s Pain Management: A Practical Guide for Clinicians. 35-48.
- [6]Jóźwiak-Bebenista, M., and Nowak, J.. Paracetamol: mechanism of action, applications and safety concern. Acta poloniae pharmaceutica, 2014; 71(1) 11-23.
- [7]Hylands-White, N., Duarte, R., and Raphael, J. An overview of treatment approaches for chronic pain management. Rheumatology International, 2017; 37.
- [8]Olufunmilayo, O., Adeyemi, S., and Okpo, O. The analgesic effect of the methanol extract of Acanthus montanus; Journal of ethnopharmacology 2004; 90 (1) 45–48.
- [9]Quintans-Júnior, L., Moreira, J., Pasquali, M., Rabie, S., Pires, A., Schröder, R., Rabelo, T., Santos, J., Lima, P., Cavalcanti, S., et al. Antinociceptive Activity and Redox Profile of the Monoterpenes (+)-Camphene, p-Cymene, and Geranyl Acetate in Experimental Models. ISRN Toxicol. 2013.
- [10]Moriasi, G., Ireri, A., Nelson, E., and Ngugi, M.. In vivo anti-inflammatory, anti-nociceptive, and in vitro antioxidant efficacy, and acute oral toxicity effects of the aqueous and methanolic stem bark extracts of Lonchocarpus eriocalyx (Harms.) Heliyon, 2021; 7(5), e07145.
- [11]Nogueira, C., Quinhones, E., Jung, E., Zeni, G., and Rocha, J. Anti-inflammatory and antinociceptive activity of diphenyl diselenide. Inflammation research, 2003; 52(2), 56-63.
- [12]Zakaria, Z., Abdul Rahim, M., Roosli, R., Mohd Sani, M., Omar, M., Othman, F., … and Abdul Kadir, A. Antinociceptive activity of methanolic extract of Clinacanthus nutans leaves: Possible mechanisms of action involved. Pain Research and Management, 2018.
- [13]Silva, R., Lima, N., Lopes, A., Vasconcelos, C., de Mesquita, J., de Mesquita, L., … and Garcia, J. Antinociceptive activity of Borreria verticillata: In vivo and in silico studies. Frontiers in pharmacology, 2017; 8, 283.
- [14]Qureshi, S. Ethnomedicinal Uses, Phytochemistry and Pharmacology of Cissampelos pareira: A Review. 2017.
- [15]Kokwaro, J. Medicinal plants of east Africa. University of Nairobi press. 2009.
- [16]Vierra, R., and Simon J. Chemical characterization of Ocimum gratissimum found in the market and used in Traditional medicine in Brazil. Journal of Economic Botany, 2000; 20, 5–6.
- [17]Nweze, E., Okafor, J., and Njoku, O. Antimicrobial activities of methanol extracts of Trema guineensis (Schumm and Thorn) and Morinda Lucida Benth used in Nigeria herbal medicinal practice. Journal of Biological Research and Biotechnology. 2004; 2, 36–39.
- [18]Vardhanabhuti, B., and Ikeda, S. Isolation and characterization of hydrocolloids from monoi (Cissampelos pareira) leaves. Food hydrocolloids, 2006; 2, 885-891.
- [19]Pérez, C., and Anesini, C. In vitro antibacterial activity of Argentine folk medicinal plants against Salmonella typhi. J Ethnopharmacol, 1994; 44, 41-46.
- [20]Matasyoh, L., Matasyoh, J., Wachira, F., Kinyua, M., Muigai, A., and Mukiama, T. Antimicrobial activity of essential oils of Ocimum gratissimum L. From different populations of Kenya. African journal of traditional, complementary, and alternative medicines: AJTCAM, 2008; 5(2), 187–193.
- [21]World Health Organization. World Health Organization on good herbal processing practices (GHPP) for herbal medicines. 2017.
- [22]Abubakar, A., and Haque, M. Preparation of Medicinal Plants: Basic Extraction and Fractionation Procedures for Experimental Purposes. Journal of pharmacy & bioallied sciences, 2020; 12(1), 1–10.
- [23]Ali Karagöz, F., Artun, G., Gülay, M., Sezin, A., Şükran, K., and Nurhayat S. In vitro evaluation of antioxidant activity of some plant methanol extracts, Biotechnology and Biotechnological Equipment, 2015; 29 (6), 1184-1189.
- [24]Anokwuru, C., Anyasor, G., Ajibaye, O., Fakoya, O., and Okebugwu, P. Effect of extraction solvents on phenolic, flavonoid and antioxidant activities of three nigerian medicinal plants. Nature and Science, 2011; 9(7), 53-61.
- [25]National Research Council. (2010). Guide for the Care and Use of Laboratory Animals. National Academies Press.
- [26]Vogel, H. Drug discovery and evaluation pharmacological assays. Springer-Verlag Berlin Heidelberg New York, 2002; 1408: 2-716.
- [27]World Health Organization. General guidelines for methodologies on research and evaluation of traditional medicines. World Health Organization, Geneva, Switzerland, 2000.
- [28]Lilienblum, W., Dekant, W., Foth, H., Gebel, T., Hengstler, J., Kahl, R. and Wollin, K. Alternative methods to safety studies in experimental animals: role in the risk assessment of chemicals under the new European Chemicals Legislation (REACH). Archives of toxicology, 2008; 82(4), 211-236.
- [29]Oecd, T. Acute Oral Toxicity: Up-and-Down Procedure. OECD Guidelines for the Testing of Chemicals, Section, 2008; 4, 1-27.
- [30]Gupta, S., George, M., Singhal, M., Sharma, G. N., and Garg, V. Leaf extract of murraya koenigii linn for anti-inflammatory and analgesic activity in animal models. Journal of advanced pharmaceutical technology and research, 2010; 1(1), 68–77.
- [31]Munene, M., Mwaniki, E., and Piero, M. Antinociceptive effects of methanolic extracts of Pistacia aethiopica and Warbugia ugandensis in mice. J Phytopharmacol 2020; 9 (5) 300 -307.
- [32]Hunskaar S., and Hole K. The formalin test in mice: dissociation between inflammatory and non-inflammatory pain. Pain. 1987; 30:103-114.
- [33]Couto, V., Vilela, F., Dias, D., Dos Santos, M., Soncini, R., Nascimento, C., and Giusti-Paiva A. Antinociceptive effect of extract of Emilia sonchifolia in mice. J Ethnopharmacol. 2011; 24 (2),348-53.
- [34]Maina, S., Kelvin, K., Maina, B., Muriithi, J., Kiambi, J., Umar, A., and Piero, M. Antinociceptive properties of dichloromethane: methanol leafand root bark extracts of Carissa edulis in rats. J Phytopharmacol, 2015; 4(2), 106-112.
- [35]Mworia, J., Gitahi, S., Juma, K., Njagi, J., Mwangi, B., Aliyu, U., … and Ngeranwa, J. Analgesic potential of acetone leaf extract of Caesalpinia volkensii Harms in mice. Pharm Anal Acta, 2015; 6, 450.
- [36]Nthiga, P., Kamau, J., Safari, V., Ngugi, M and Mburu, D. Antinociceptive Activity of Methanol Stem Bark Extracts of Harrisonia Abyssinica Oliv and Landolphia Buchananii (Hallier F.) Stapf in Mice Model. Journal of Pain and Relief 2016; 5: 253.
- [37]Koech, S., Odhiambo, R., Michael, N., Ireri, M., Ngugi, P., and Njagi. M., Analgesic Activity of Dichloromethanolic Root Extract of Clutia abyssinica in Swiss Albino Mice. Natural Products Chemistry and Research. 2017; 05.
- [38]Mworia, J., Kibiti, C., Ngeranwa, J., and Ngugi, M. Analgesic potential of dichloromethane leaf extracts of Eucalyptus globulus (Labill) and Senna didymobotrya (Fresenius) in mice models. Journal of Herbmed Pharmacology, 2020; 9(4), 391-399.
- [39]He, P., and Aga, D. Comparison of GC-MS/MS and LC-MS/MS for the analysis of hormones and pesticides in surface waters: Advantages and pitfalls. Analytical Methods. 2019.
- [40]Pond, S., Tozer, T. First-Pass Elimination Basic Concepts and Clinical Consequences. Clin Pharmacokinet, 1984; 9, 1–25.
- [41]Frutuoso, V., Monteiro, M., Amendoeira, F., Almeida, A., Nascimento, D., Bérenger, A., … and Castro-Faria-Neto, H. Analgesic and anti-inflammatory activity of the aqueous extract of Rheedia longifolia Planch and Triana. Memorias do instituto oswaldo cruz, 2007; 102(1), 91-96.
- [42]Veronica, S., Cheruiyot, K., Bosibori, M., Munene, I., Murugi, J., and Piero, M. Antiinflammatory, analgesic and antipyretic effects of dichloromethane stem bark extract of Acacia mellifera. J. Phytopharmacol, 2017; 6(4), 239-246.
- [43]Maina, B., Maina, S., Muriithi, J., Kiambi, J., Umar, A., et al. Antinociceptive Properties of Dichloromethane: Methanolic Leaf Extracts of Caesalpinia volkensii and Maytenus obscura in Animal Models. J Pain Relief, 2015; 4,191.
- [44]Safari, V., Kamau, J., Nthiga, P., Ngugi, M., Orinda, G., et al. Antipyretic, Anti-inflammatory and Analgesic Activities of Aqueous Leaf Extract of Aloe volkensii in Albino Mice. Med Aromat Plants 2016; 5, 240.
- [45]Ong, H., Mohamad, A., Makhtar, N., Khalid, M., Khalid S. and Perimal, E. Antinociceptive activity of methanol extract of Acmella uliginosa (Sw.) Cass. 2011; 12,4-6.
- [46]Anderson, B., Paracetamol (acetaminophen): mechanisms of action. Paediatr Anaesth, 2008; 18(10):915–921.
- [47]Tanko, Y., Magaji, G., Yerima, M., Magaji, R., and Mohammed, A. Anti-nociceptive and anti-inflammatory activities of aqueous leaves extract of Ocimum Gratissimum (Labiate) in Rodents. African Journal of Traditional, Complementary and Alternative Medicines, 2008; 5(2), 141-146.
- [48]Shojaii, A., Motaghinejad, M., Norouzi, S., and Motevalian, M. Evaluation of Anti-inflammatory and Analgesic Activity of the Extract and Fractions of Astragalus hamosus in Animal Models. Iran J Pharm Res, 2015; 14(1):263-269.
- [49]Liapi, C., Anifantis, G., Chinou, I., Kourounakis, A., Theodosopoulos, S., and Galanopoulou, P. Antinociceptive properties of 1, 8-cineole and β-pinene, from the essential oil of Eucalyptus camaldulensis leaves, in rodents. Planta medica, 2007; 73(12), 1247-1254.
- [50]Guimarães, A., Quintans, J., and Quintans‐Júnior, L. Monoterpenes with analgesic activity—a systematic review. Phytotherapy research, 2013; 27(1), 1-15.
- [51]de Santana, M., Guimarães, A., Chaves, D., Silva, J., Bonjardim, L., Lucca Júnior, W., … and Villarreal, C. The anti-hyperalgesic and anti-inflammatory profiles of p-cymene: Evidence for the involvement of opioid system and cytokines. Pharmaceutical Biology, 2015; 53(11), 1583-1590.
- [52]Bonjardim, L., Cunha, E., Guimarães A, et al. Evaluation of the anti-inflammatory and antinociceptive properties of p-cymene in mice. Z Naturforsch CJ Biosci, 2012; 67(1-2):15-21.
- [53]Hotta, M., Nakata R., Katsukawa, M., Hori, K., Takahashi, S., and Inoue H. Carvacrol, a component of thyme oil, activates PPARalpha and gamma and suppresses COX-2 expression. J Lipid Res, 2010; 51(1):132-139.
- [54]Ji, J., Zhang, R, Li, H., Zhu, J., Pan, Y., and Guo, Q. Analgesic and anti-inflammatory effects and mechanism of action of borneol on photodynamic therapy of acne. Environ Toxicol Pharmacol, 2020; 75:103329.
- [55]Monteiro, É., Chibli, L., Yamamoto, C., Pereira, M., Vilela, F., Rodarte, M., de Oliveira Pinto, M., da Penha Henriques do Amaral, M., Silvério, M., de Matos Araújo, A. and da Luz André de Araújo, A., Antinociceptive and anti-inflammatory activities of the sesame oil and sesamin. Nutrients, 2014; 6(5), pp.1931-1944.
- [56]Singh, S., Taneja, M., and Majumdar, D. Biological activities of Ocimum sanctum L. fixed oil—An overview. Indian Journal of Experimental Biology, 2007; 45, 403–412.
- [57]Emin, J., Oliveria, A., and Lappa, A., Pharmacological evaluation of anti-inflammatoyr activity of citrus bioflavonoid, hespridin and isoflavonoids duartinand claussequinone in rats and mice. J pharm pharmacology, 1994; 46118-122.
- [58]Alcaraz, M., and Houli R. Action of flavonoids and the novel anti-inflammatory flavone, Hyperlactin-8-Glucoside on prostaglandin biosynthesis and inactivation. Journal of biochemistry pharmacology, 1985; 34(14) 2477-82.
- [59]Tjølsen, A., and Hole, K. Animal models of analgesia. In The pharmacology of pain. Springer, Berlin, Heidelberg, 1997; pp. 1-20.
- [60]Aouey, B., Samet, A., Fetoui, H., Simmonds, M., and Bouaziz, M. Antioxidant, anti-inflammatory, analgesic and antipyretic activities of grapevine leaf extract (Vitis vinifera) in mice and identification of its active constituents by LC–MS/MS analyses. Biomedicine and pharmacotherapy, 2016; 84 1088-1098.
- [61]Mansourabadi, A., Sadeghi, H., Razavi, N., and Rezvani, E. Anti-inflammatory and analgesic properties of salvigenin, Salvia officinalis flavonoid extracted. Advanced Herbal Medicine, 2016; 2(1), 31-41.
- [62]Nijveldt, R., Van Nood, E., Van Hoorn, D., Boelens, P., Van Norren, K., and Van Leeuwen, PA. Flavonoids: a review of probable mechanisms of action and potential applications. The American journal of clinical nutrition, 2001; 74(4), 418-425.
- [63]Goyal, M., Ghosh, M., Nagori, B., and Sasmal, D. Analgesic and anti-inflammatory studies of cyclopeptide alkaloid fraction of leaves of Ziziyphus nummularia. Saudi journal of biological sciences, 2013; 20(4), 365-371.